Laboratory for Zero-Carbon Energy, Tokyo Institute of Technology
Prof. Junichiro Otomo
How far have we come on the road from low carbon to decarbonization? The global climate change problem has gone beyond mere energy and environmental issues and is reaching the stage of transforming the industrial structure. Meanwhile, the international community has set a goal of limiting the increase in global average temperature to 1.5 degrees Celsius above pre-industrial levels, but it is not clear at this point whether that goal can be achieved. However, despite various obstacles, the world as a whole is moving forward along a major trend. Japan's efforts to become carbon neutral (CN) have also been remarkably active over the past decade, and the Tokyo Tech GXI plays an important role as a hub for Green Transformation (GX) research, serving as a point of contact between companies and universities.
The measures to address climate change are a major opportunity for change for Japan, which relies heavily on energy imports. It is also an opportunity for value creation in terms of energy cost reduction and security. A decade ago, solar cells were synonymous with extremely expensive power generation devices, but this situation has changed dramatically in the past decade, and they have been transformed into a major low-cost power source on a global scale. Although the integrated costs of deploying power grids and including energy storage for the introduction of new energy sources will rise, there will be no change in the currents that are changing society as a whole, incorporating a variety of new energy sources in a major shift from conventional wisdom.
Considering the above major trends surrounding energy, it is an important approach to promote research and collaborative activities that extend beyond basic research on energy conversion systems to their introduction into society. Considering future innovations in energy conversion technology, it is becoming increasingly important to develop chemical reactions and materials involved in the energy conversion process, and to design and integrate energy systems based on new fundamental knowledge of these reactions and materials to provide a path for introducing new technologies into society. As shown in Figure 1, we believe that bridging the four layers (materials/reaction processes, devices/systems, energy networks, and society) with design parameters for energy/social transformation can provide an integrated perspective and vision for the future. We refer to our work as cost engineering, and we conduct techno-economic minanalyses of energy devices/systems such as hydrogen production, fuel cells, and electrolytic synthesis, as well as design and evaluation of new systems [1,2].
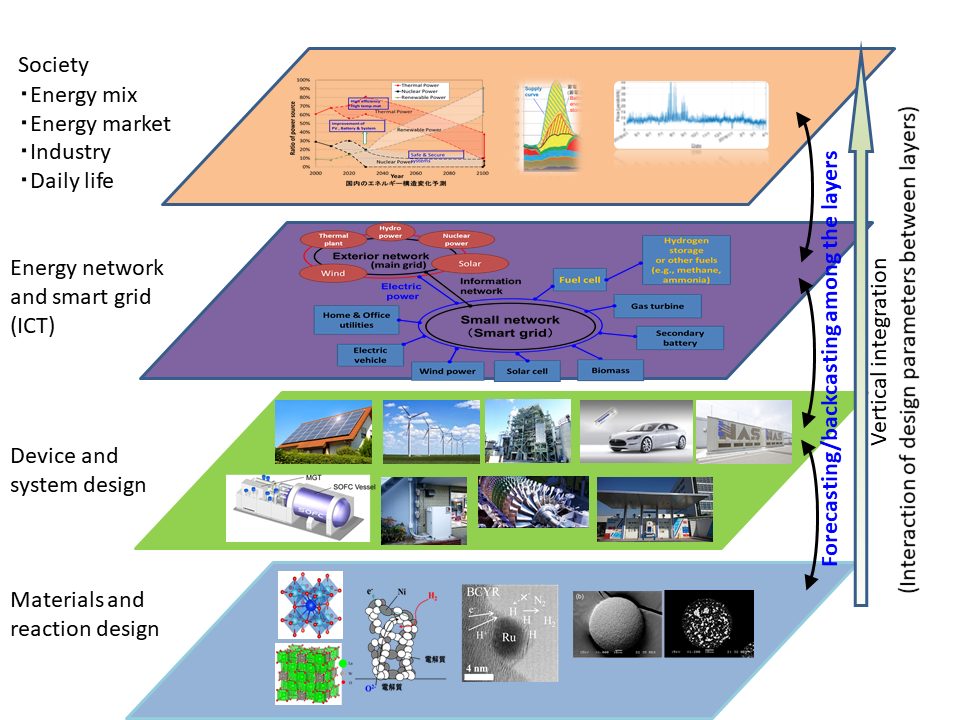
Figure 1: Four layers and integrated perspectives and visions for energy/social transformation
The main points of the GX research are summarized below.
Promotion of basic research on new energy and material circulation
Evaluation of a series of micro phenomena to macro systems, including materials, reactions, and systems.
Approach to future energy systems from an integrated techno-economic perspective
Exploration of energy systems from a broad perspective, from basic research to technological evolution and social introduction.
As individual GX elemental technology research, we are conducting research in the following four main fields: Development of high-efficiency fuel cells and electrolysis cells [3], Electrochemical synthesis by electrochemical enhancement [4], Chemical loop method supporting carbon cycle [5], and 4) Techno-economic analysis by cost engineering as mentioned above.
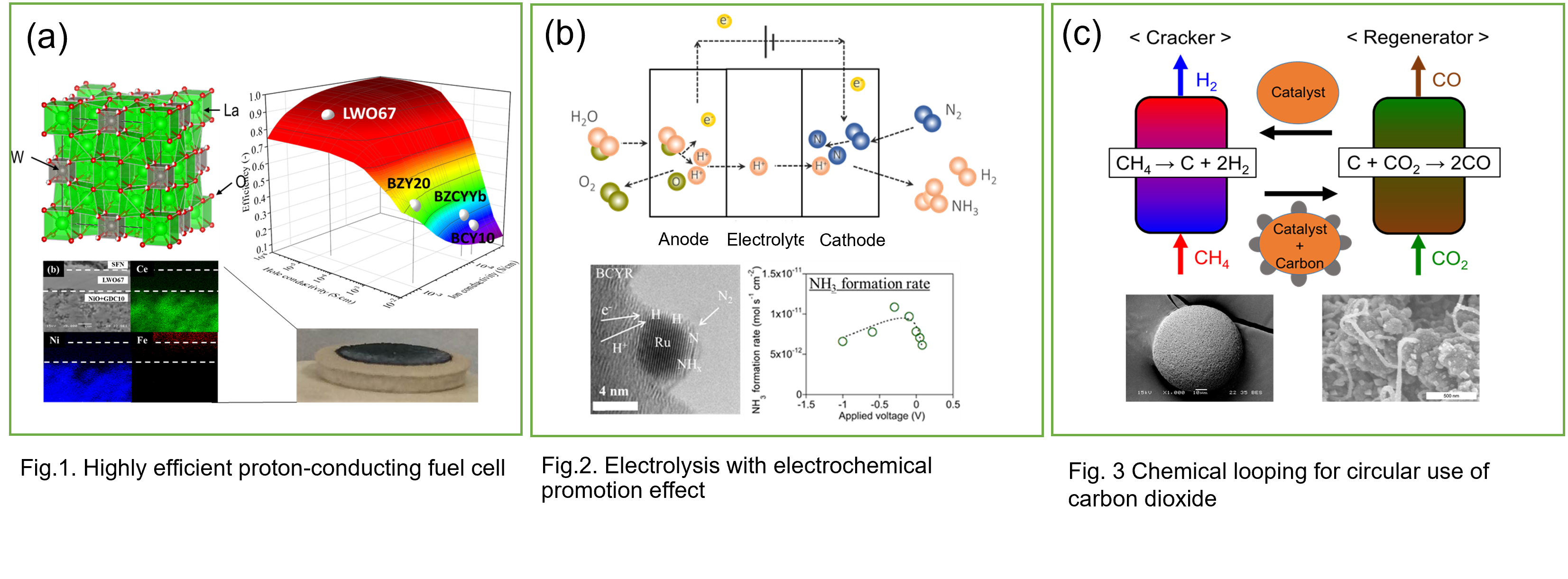
Figure 2 : GX Basic Research (a) High-efficiency proton-conducting ceramic fuel cell; (b) Electrochemical synthesis of ammonia, etc. by electrochemical promotion effect; (c) Chemical loop method using carbon cycle
Technological economics through cost engineering: An example of analysis of Solid Oxide Fuel Cells (SOFC) is presented [6]. The analysis, which covers everything from material properties to device and system design, shows a path for technological development to reach conventional grid power costs by advancing the calculation of power generation costs based on future technological innovations.
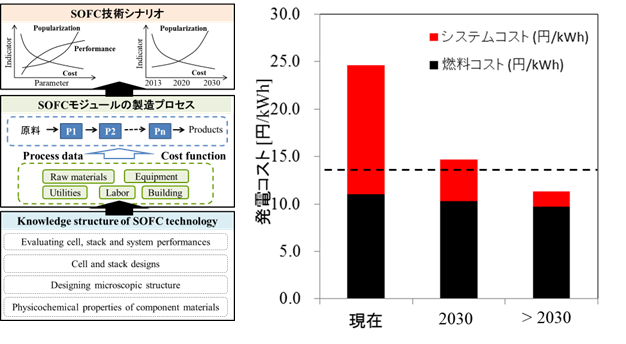
Figure 3 : Cost Engineering of SOFC
In addition, at the Science Tokyo GXI experimental building (Ookayama Lab), research is being conducted on the development of iron-based metal oxide carrier particles, which are the circulating media for the three-tower carbon recirculation chemical loop method, and on reactor design[7]. min(1) Carbon dioxide is separated from biomass and other carbon-containing fuels in a reduction tower through the reduction of carrier particles, min; (2) pure hydrogen is produced by reacting the reduced carrier particles with water vapor in a hydrogen production tower; and (3) heat is extracted and used for power generation by completely oxidizing the oxidized carrier particles with air. This process is called polygeneration, and research is being developed to design actual equipment through industry-academia-government collaboration.
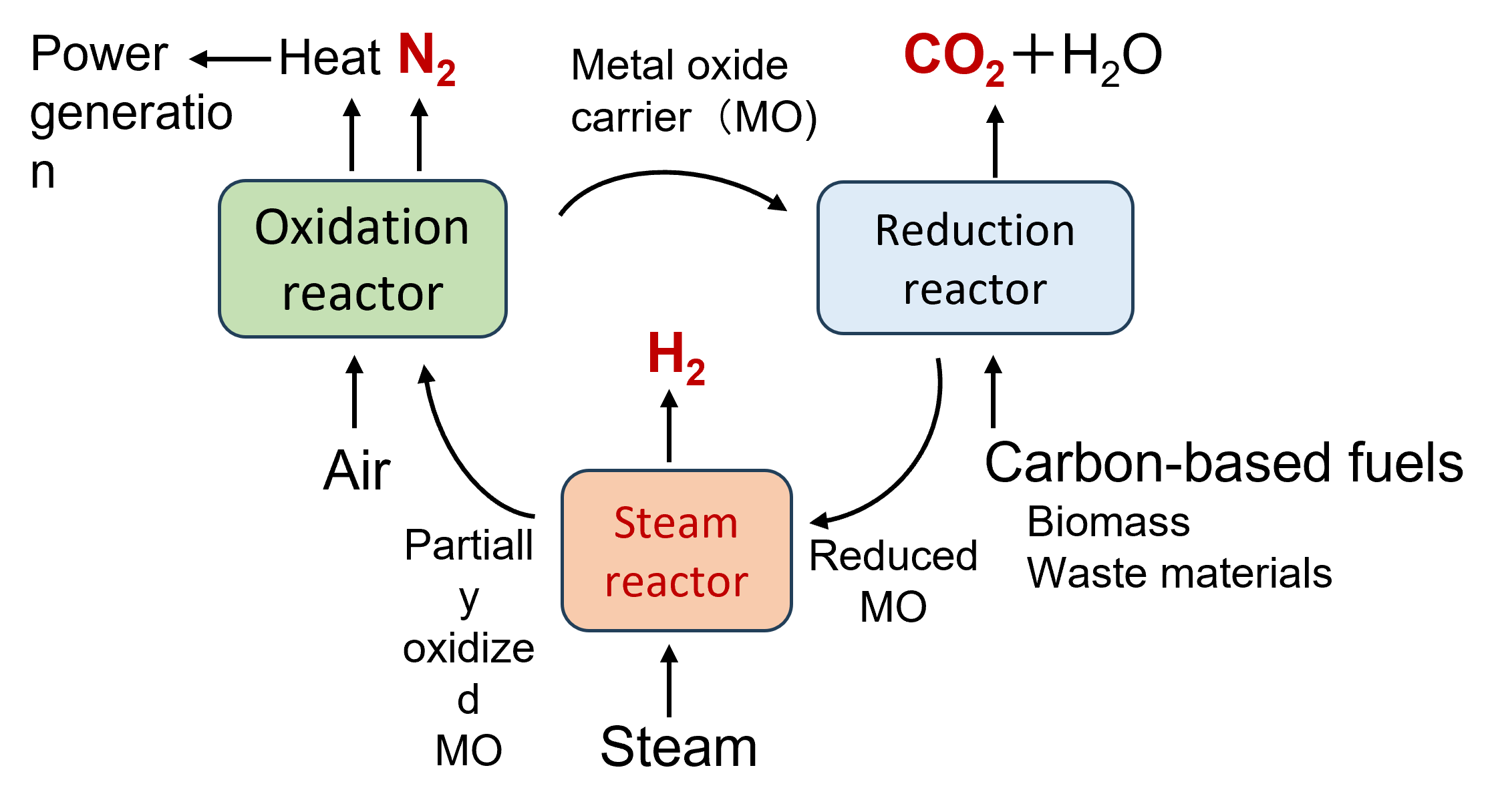
Figure 4 : Three-tower polygeneration chemical loop system
In promoting GX, it is necessary to draw scenarios linking technology and society, and to specify the technological issues involved in introducing GX to society. At present, we feel that there is a large distance between researchers who are responsible for technological innovation and those who are responsible for social innovation. In addition to promoting industry-academia-government collaboration, effective collaboration between natural science and engineering, as well as social science, is now required.
References
[1] Shun Yamate and Junichiro Otomo
Energy Conversion and Management, 301 (2024) 118016.
DOI: https://doi.org/10.1016/j.enconman.2023.118016
[2] Taihei Yamamura, Taisuke Nakanishi, Jihan Lee, Shun Yamate, Junichiro Otomo
Energy & Fuels, 36(17) (2022) 9745.
DOI information: https://doi.org/10.1021/acs.energyfuels.2c00910
[3] Julián A. Ortiz-Corrales, Hiroki Matsuo, and Junichiro Otomo
Journal of The Electrochemical Society, 170 (2023).
DOI: https://iopscience.iop.org/article/10.1149/1945-7111/ad1631
[4] M. Okazaki and J. Otomo
ACS Omega, 8(43) (2023) 40299
DOI information: https://pubs.acs.org/doi/10.1021/acsomega.3c04478
[5] Shu Hikima, Martin Keller, Hiroki Matsuo, Yoshio Matsuzaki, Junichiro Otomo
Chemical Engineering Journal, 417 (2021) 128012.
https://doi.org/10.1016/j.cej.2020.128012
[6] Junichiro Otomo, Junya Oishi, Kenya Miyazaki, Shintaro Okamura and Koichi Yamada
International Journal of Hydrogen Energy, 42 (30), (2017) 19190.
Available at: http://dx.doi.org/10.1016/j.ijhydene.2017.06.031
[7] Junichiro Otomo
Journal of the Japan Petroleum Institute, 60(1) (2022) 1.
DOI information: https://doi.org/10.1627/jpi.65.1